Mass Spectrometry
- Direct-Push Technologies
- Explosives
- Fiber Optic Chemical Sensors
- Gas Chromatography
- Geophysical Methods
- High-Resolution Site Characterization (HRSC)
- Immunoassay
- Infrared Spectroscopy
- Laser-Induced Fluorescence
- Mass Flux
- Mass Spectrometry
- Open Path Technologies
- Passive (no purge) Samplers
- Test Kits
- X-Ray Fluorescence
Introduction
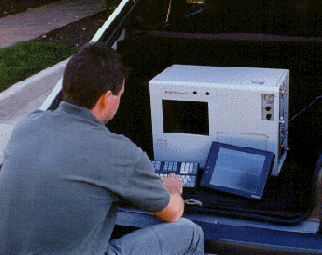
The first general application of molecular mass spectrometry occurred in the early 1940s in the petroleum industry for quantitative analysis of hydrocarbon mixtures in catalytic crackers.
Coupling mass spectrometers with gas chromatography (GC) systems allows separation and subsequent determination of components of highly complex mixtures with a high degree of certainty. Similar compounds may be retained for different lengths of time on the GC column, allowing separate identification and quantitation, even if the two compounds, or fragments of compounds, have similar mass to charge (m/z) ratios. Retention time thus provides a secondary source of identification. For a discussion of identification by retention time see Gas Chromatography.
Recently, manufacturers of mass spectrometers, particularly spectrometers coupled with GC systems, have significantly reduced their overall size and have increased durability. These changes allow what was once a laboratory bench top instrument to be portable (or transportable), and sufficiently rugged to perform field analysis.
Typical Uses
Mass spectrometry has applications in site characterization, for the analysis of soil, water and gas matrices. Some MS systems have been "hardened" for military use. These are usually hand portable instruments used for the detection of airborne chemical warfare agents.
In the environmental field, mass spectrometers are often used as detectors for GC systems. Some field GC/MS may be capable of the same analyses as fixed laboratory instruments. U.S. Environmental Protection Agency (EPA) SW-846 methods may be possible with some field GC/MS instruments. SW-846 methods that could be adapted for field use include:
- Semivolatile Organic Compounds by Gas Chromatography/Mass Spectrometry (GC/MS) Method 8270D
- Volatile Organic Compounds by Gas Chromatography/Mass Spectrometry (GC/MS) Method 8260B
- Semivolatile Organic Compounds (PAH and PCBs) in Soils/Sludges and Solid Wastes using Thermal Extraction/Gas Chromatography/Mass spectrometry TE/GC/MS Method 8275A
- Parent and Alkyl Polycyclic Aromatics In Sediment Pore Water By Solid-Phase Microextraction and Gas Chromatography/Mass Spectrometry in Selected Ion Monitoring Mode Method 8272
A SW-846 Method that addresses field analysis using MS, but does not require coupling to a GC system is:
- Volatile Organic Compounds in Water, Soil, Soil Gas and Air by Direct Sampling Ion Trap Mass Spectrometer (DSITMS) Method 8265
For descriptions of how samples may be prepared for introduction or introduced into the GC/MS system refer to Gas Chromatography.
Theory of Operation
As an analyte is introduced into the mass spectrometer, it is ionized in an electron stream produced by an ion source, typically a metallic filament under an applied voltage. In the case of GC/MS, the analyte eluting from the GC column is directed into the ion source of the mass spectrometer through a heated interface. The loss of an electron by the analyte during ionization generates the molecular ion. The charged molecular ion has the same molecular weight as the analyte molecule. The electron stream is generally held at a higher energy level than is necessary to produce the molecular ion. Excess energy from the beam further excites the molecular ion, that subsequently fragments to daughter ions with lower m/z ratios.
The positive ions produced by the electron stream are then focused toward the detector, the mass analyzer. These ions are differentiated according to their mass-to-charge ratios.
The mass sorted ions are detected by an electron multiplier and the resulting signal is sent to a data system for processing. A display of the electron multiplier signal generated by the sorted molecular ions is displayed as the mass spectrum.
In the case of a coupled GC/MS, one type of data display is an integrated sum of signals generated by the molecular ions plotted as a function of elution time from the GC column. This representation is a total ions current (TIC) chromatogram. Mass spectra can be extracted from each chromatographic peak of the TIC.
The mass spectrum is in the form of a bar graph that relates the relative intensity of mass peaks to their mass-to-charge ratio. The largest peak in each spectrum is termed the base peak. The heights of the remaining peaks are computed as a percentage of the base peak height. The spectrum may be compared to a spectral library for identification of the compound based on fragmentation pattern and peak ratios.
The individual TIC and ion peak intensities are directly related to the concentration of analyte in the sample extract making GC/MS an extremely powerful analytical tool for positive identification and quantitation of organic compounds.
System Components
Mass spectrometers consist of four basic components; ion sources, sample inlet systems, mass analyzers, and a transducer. A brief description of various types of each component is presented below.
Ion Sources
The starting point for a mass spectrometric analysis is the formation of gaseous analyte ions. Ionization methods fall into two categories: gas phase sources and desorption sources. Gas phase ionization relies on first vaporizing the analyte then ionizing it. Desorption sources ionize the analyte in a solid or liquid state [list of ionization sources].
Sample Inlet Systems
The purpose of the inlet system is to permit the introduction of a representative sample into the ion source. Types of inlets include batch inlets, direct probe inlets, and chromatographic inlets. In the instances when mass spectrometers are used for environmental analysis as detectors for GC systems, the flow rate from capillary chromatographic columns is generally low enough that the column output can be fed directly into the ionization chamber of the mass spectrometer. For packed or megabore capillary columns, a jet separator is used to eliminate most of the carrier gas from the analyte.
Mass Analyzers
The function of the mass analyzer is analogous to that of the grating in an optical spectrometer. In mass spectrometers, dispersion is based upon the mass-to-charge ratios of the analyte ions rather than upon the wavelength of photons. Mass spectrometers are therefore categorized based on the type of mass analyzer in the instrument.
Two types of mass analyzers are typically used for GC/MS analysis: (1) the quadrupole mass analyzer, (2) magnetic sector analyzer and (3) ion trap mass analyzer. The quadrupole MS interfaced with capillary-column GC, is the most commonly used in an environmental laboratory and field analysis. The sample extract is injected onto the capillary column on the GC, where the individual compounds in the complex mixture are separated. The individual compounds elute through the column at different rates into the MS for detection.
The second type of mass analyzer typically used in mass spectrometers is the magnetic sector. Magnetic sector mass spectrometers, also known as high resolution mass spectrometers, are significantly more sensitive than the quadrupole. For example, magnetic sector spectrometers detect dioxins at parts per trillion (ppt) levels in soil and parts per quadrillion (ppq) in water. Typically, high resolution MS is not used for field-based environmental analysis because of size and stability requirements of the instrument.
A description of ion trap mass spectroscopy is provided in the U.S. Department of Energy Innovative Technology Summary Report Direct Sampling Ion Trap Mass Spectrometry (DSITMS) 1998.
Transducers
Like an optical spectrometer, a mass spectrometer contains a transducer that converts the beam of ions into an electrical signal that can be processed, stored in computer memory, and presented in graphical form. Transducers commercially available for mass spectrometers include electron multipliers and the Faraday Cup. The electron multiplier is the transducer of choice for most spectrometers. Less common detection systems include photographic plates and scintillation counters.
Data Acquisition and Data Handling Systems
GC/MS units require powerful computing systems capable of collating the data from the transducer, producing TICs and mass spectra, and interpreting the data for each compound encountered in analysis. To identify a compound, the computer looks for a match between the results of analysis of the compound and mass spectral data stored in a library. A mass spectral library may contain information for many thousands of compounds. The computer identifies a compound on the basis of the best spectral match. Mass spectra should be reviewed by an experienced spectroscopist as a quality control measure, to ensure correct interpretation by the system.
Mode of Operation
Mass spectrometers are operated under high vacuum to remove atmospheric gases that would affect the ionization process by combining with target analytes and producing unwanted ionic species. All MS have safety devices so that ion source cannot become operational until high vacuum is attained. Because most MS are operated in conjunction with a gas chromatography, the removal of carrier gas is also critical.
Computers and microprocessors are integral in modern mass spectrometers. A characteristic of a mass spectrum is the wealth of structural data that it provides. For structural determination of a molecule, the heights and mass-to-charge ratios of each fragmentation peak in a spectrum are determined, stored, and displayed. Because the amount of information is so large, rapid acquisition and processing is essential. The microprocessor is essential for mass spectrometric data acquisition and manipulation. Several instrumental variables are controlled and monitored during data collection. First, the computer serves as the instrument controller. Operating parameters are set through communication via the keyboard. Secondly, the computer controls programs responsible for data manipulation and output.
The digitized ion-current signal is processed prior to display. The peaks are normalized, that is, the height of each peak relative to a reference peak is calculated. The largest peak in the spectrum, the base peak, is arbitrarily assigned a peak height of 100 (sometimes 1000). The mass to charge (m/z) value for each peak must also be determined. This assignment is frequently based on the time of the peak's appearance and the scan rate. Data are acquired as intensity versus time during a controlled scan of the magnetic or electric fields. To ensure that the MS system is assigning the correct mass to ions, the instrument is "tuned" using a standard solution such as 4-bromofluorobenzene or perfluorotri-n-butylamine for VOC analysis, or decafluorotriphenylphosphine for SVOC analysis. If the system identifies and attributes the correct masses in the correct proportions to ions resulting from the fragmentation of a tuning solution, the instrument can then be further calibrated so that quantitative analysis can begin. The computer is programmed to recognize and use the peaks of the calibration standard as references for mass assignments.
Selected Ion Monitoring (SIM) Mass spectrometry has been applied to the quantitative determination of one or a small number of components of complex organic systems that are encountered in studies of environmental matrices. In SIM mode, analyses are performed by passage of the sample through a chromatographic column and into the spectrometer, with the spectrometer set at the mass-to-charge (m/z) ratios of the fragments of the target compounds, rather than scanning a wide range of masses. In this type of analysis, the mass spectrometer serves as a sophisticated selective detector. Extremely low detection limits can be achieved in SIM mode, and as the instrument is looking for fewer fragments, matrix interference can be reduced. SIM is most usually used when the identities of the contaminants of concern are known, and extremely low detection limits are required.
Full Scan Mode is most generally used for field analyses and the MS is programmed to scan a wide range of masses, often from m/z 50 through m/z 400. Full scan mode is used to identify unknowns in a sample, and does not have the great sensitivity of SIM. In full scan mode, the instrument is gathering data over a wide range and very large number of m/z values and cannot perform the greater numbers of scans per second that would give very low detection limits.
Target Analytes
- Semi-volatile organic compounds including pesticides, polychlorinated biphenyls (PCBs) and polyaromatic hydrocarbons (PAHs).
- Volatile organic compounds, including benzene, ethylbenzene, toluene and xylene (BTEX), chlorinated species such as trichloroethene, dichloroethenes, tetrachloroethene.
Performance Specs
Performance specifications include information on detection limits, sample throughput, precision, accuracy, comparability to other methods, ease of deployment, ability of the instrument to complete a required number of samples. The performance specifications for the Inficon "HAPSITE" instrument are included in the EPA's Environmental Technology Verification Report available at the end of this document.
A useful brief overview of the operation of a portable GC/MS system in the field is provided by Los Alamos National Laboratory. This guide gives clear examples of TICs and mass spectra.
Detection Limits
Part per billion detection limits have been reported for quadrupole mass spectrometers and ion trap mass spectrometers. Magnetic sector mass spectrometers, also known as high resolution mass spectrometers, are significantly more sensitive than the quadrupole. For example, magnetic sector spectrometers detect dioxins at parts per trillion (ppt) levels in soil and parts per quadrillion (ppq) in water.
Quality Control
Ensuring that the data generated is of a known quality is vital to the usefulness of those data. Quality control (QC) measures take several forms. They should be performed in the field, during sample analysis, and after sample data have been collected. The type and extent of QC necessary will vary according to the test to be performed and the data quality objectives of the project. A much higher level of QC is necessary to produce defensible data that will be used alone to support specific decisions than to produce screening data that will not be used alone to support decision-making. A fuller discussion of QC for field analytical systems is presented in "Using Dynamic Field Activities for On-Site Decision Making: A Guide for Project Managers" EPA/540/R-03/002 May 2003. In addition, a comprehensive list of QC samples and the information they provide is available.
The SW-846 "8200" series of methods provides guidance for calibrating GC/ MS instruments. Instrument manufacturers will also provide guidance on the calibration of their particular systems.
Method blanks are periodically analyzed to monitor laboratory and instrument-induced contaminants. A method blank must not contain any analyte in a concentration higher than the practical quantitation limit.
Matrix spike and matrix spike duplicate samples are analyzed to evaluate the efficiency of the sample preparation, precision of the analysis, and matrix effect.
Laboratory control samples are used to evaluate the accuracy of the analysis. The control samples are obtained from outside sources and contain known amounts of analytes in what is generally an artificial matrix to avoid the matrix effects common to "real" site matrices. The values obtained by analysis of the control samples are compared with the known true values. The supplier of the control samples usually provides control limits. The results obtained should fall within the published range of acceptance values.
Advantages
- Using full scan mode, samples can be surveyed in a single analysis for the presence of a broad spectrum of organic chemical compounds.
- Field portable or transportable GC/MS analysis can be of equal quality as fixed laboratory data.
- Rapid analysis provides data that can be used to enable field decision-making, expediting cleanup or characterization.
- Mass spectrometry can provide definitive compound identification.
Limitations
- Instrumentation is expensive.
- Instrumentation operation requires a higher degree of expertise than most other instrumentation.
- Full scan mode for the full range of compounds cannot produce the analytical accuracy and sensitivity possible with selected ion monitoring (SIM).
Choosing a field GC/MS or MS System
Points to consider in the choice if a field GC/MS or MS instrument:
- Field portable, or transportable? A portable GC/MS should be self-contained, need no exterior power source, weigh less than 40 lbs and be easily portable. Transportable GCs are systems that can be packed into a crate for shipping and transported by truck or van. These instruments are not hand portable, and generally require operation from the back of a van, or in a trailer.
- Durability. Is the GC/MS capable of handling adverse conditions? Can it operate in conditions of high humidity, high and low ambient temperatures? Will the operator be able to use the instrument when wearing mandated personal protective equipment such as gloves? Is the instrument rugged?
- Ease of operation. Are the instrument's controls easy to use when the operator's hands are cold? Are the panels that display results or operating parameters, easy to read, even in poor lighting conditions?
- Operator training. Does the manufacturer provide "hands-on" training on the system, or do they provide instructional videos or DVDs?
- Detection limits. Can the instrument achieve the detection limits required, or can the operating conditions be modified to do so?
- Turn-around. What kind of sample throughput can be expected? Is the introduction of the sample into the system straightforward?
- Sample delivery options. Does the instrument support a variety of sampling techniques, such as loop and syringe injection, sampling by probe, or solvent phase micro-extraction (SPME)?
Verification/Evaluation Reports
Verification of the performance of site characterization and field analytical technologies is conducted through a variety of programs. Evaluation and verification reports from EPA's Superfund Innovative Technologies Evaluation (SITE) Measuring and Monitoring Program and EPA's Environmental Technology Verification Program (ETV) program are provided below.
Superfund
Innovative Technologies Evaluation (SITE) Measuring and Monitoring Program
The SITE Demonstration Program encourages the development and implementation of innovative treatment technologies for (1) remediation of hazardous waste sites and (2) monitoring and measurement. In the SITE Demonstration Program, the technology is field-tested on hazardous waste materials. Engineering and cost data on the innovative technologies are gathered so that potential users can assess the technology's applicability to a particular site. Data collected during the field demonstration are used to assess the performance of the technology, the potential need for pre- and post-treatment processing of the waste, applicable types of wastes and waste matrices, potential operating problems, and approximate capital and operating costs. The following reports from the measuring and monitoring program are available for mass spectrometry:
No reports available for this technology
EPA's
Environmental Technology Verification (ETV) Program
EPA's Environmental Technology Verification (ETV) Program verifies the performance of innovative technologies. ETV was created to substantially accelerate the entrance of new environmental technologies into the domestic and international marketplaces. ETV verifies commercialized, private sector technologies. After the technology has been tested, the companies will receive a verification report that they can use in marketing their products. The results of the testing also are available on the Internet. The following reports from the ETV program are available for mass spectrometry:
- Inficon, Inc. - HAPSITE with Headspace Sampling Accessory was verified for measurement of chlorinated volatile organic compounds in water. The verification documents available consist of a verification report (516/79pp/PDF) and verification statement (28/3pp/PDF).
Additional Information
Although all the instrumentation described in U.S. Department of Energy Innovative Technology Summary Report Direct Sampling Ion Trap Mass Spectrometry (DSITMS) 1998 may be currently available, the document provides much useful information on the basic technology. Some indication of the performance specifications that could be expected from ion trap mass spectroscopy are provided in the document.